Understanding Life Cycle Impact Assessment Process: Part I–Overview and Fate
Life Cycle Impact Assessment (LCIA) is a central element of Life Cycle Assessment (LCA) -- the point in the LCA process where potential environmental impacts of a product or service are identified and quantified.
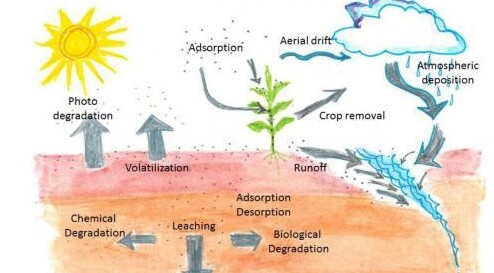
Life Cycle Impact Assessment (LCIA) is a central element of Life Cycle Assessment (LCA) -- the point in the LCA process where potential environmental impacts of a product or service are identified and quantified.
This is part one of a three-part series that reviews the three distinct phases of LCIA impact modeling (fate, exposure, and effect), and explains how they build on one another to create a usable picture of environmental impacts for use in broader analyses.
Predicting these types of consequences can be extremely complex, due to the long, interconnected pathways of interaction between environment and human activity. But decades of development have resulted in an LCIA process capable of providing reliable insights into the impacts of materials and manufacturing processes.
One of the basic challenges of LCIA is that the interaction pathways between the ecosystems and the pollutants are typically nonlinear and are affected by multiple variables. For example, the biological response of an organism might not increase in direct proportion to increases in a pollutant’s concentration – in many cases, once an inflection point is reached, the response can increase more rapidly.
Fortunately, we can draw on mathematical models to create a simplified version of reality that enables us to predict, with some amount of acknowledged uncertainty, the possible outcomes of an upstream decision. These models can mimic the natural processes the emission will go through -- and the impact it will have on human health and plant and animal species. Some of the commonly used models in LCA are USEtox (Hauschild et al., 2008) for toxicity, the IPCC model (IPCC, 2007) for climate change and EUTREND (Van Jaarsveld, 1995) for acidification.
In LCIA, impacts are modeled in three distinct phases: fate, exposure and effect (Heijungs and Wegener Sleeswijk, 1999), as shown in Exhibit 1.

- Fate modeling accounts for the characteristics of an emission and the environmental concentration it forms once released.
- Exposure modeling looks at the intake level of the emission by considering various routes and modes of intake; for ecosystems, exposure models consider the amount of the emission that becomes bioavailable
- Once exposure is assessed, effect models link this information to known toxicity data at those intake levels.
Using Temporal and Spatial Integration to Model Fate
To model fate, we begin by calculating a steady-state environmental concentration of the pollutant (Goedkoop et al., 1999). Before arriving at this steady state, the pollutant generally goes through multiple environmental mechanisms, such as transport, degradation, volatilization, sedimentation or immobilization (Exhibit 2).

The ultimate concentration is calculated by using steady-state box models where the concentration of the pollutant inside the box does not change, i.e., remains in a steady state (Exhibit 3). This figure shows a simplified version of a fate model where the steady state concentration can be calculated for a given volume if the source rate of the pollutant inflow and the pollutant residence time are known.

The advantage of this type of model is that it allows each emission to be related to a single time-integrated fate value instead of multiple values at different points in time.
In addition to this temporal integration, fate models also use spatial integration – in other words, they do not detail where the emissions take place. Typically, the only spatial information included is the type of environmental media, such as air, surface water, sea water, soil, sediment etc.
These temporal and spatial integrations are the two primary model simplifications that enable us to reduce complexity while still obtaining valid results. Newer models, however, are attempting to include more spatial details to reduce uncertainty levels. For example, the IMPACT World+ method uses the spatially differentiated USEtox toxicity model that includes 17 sub-continental zones, such as Central America, North America, Europe, Middle East and Southeast Asia (Kounina et al., 2014).
Fate models also consider at least three emission compartments (air, water, and soil) and at least four transport compartments (air, water, soil, and sediment). The transport compartments account for multimedia transport, i.e., transport from one media to another, such as air to water (as with, for example, acid rain). And since an emission can easily be distributed over multiple compartments, most emissions are linked to multiple fate factors.
In the next installment of this series, we’ll look at the next step in the LCIA process – modelling the exposure of humans and other organisms to the emissions whose fate we have calculated.
We hope you’re finding this discussion of LCIA useful; if you have questions or comments, please feel free to post them below!
For more information on impact assessment methods and models, please see the free online course on impact assessment and the free brown bag webinars offered by Earthshift Global: